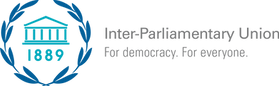
The IPU Science for Peace Schools
Topic 4 – Challenges of Fundamental Science: Ethics and International Collaboration
Speaker: Dr. Michael Doser (Senior Research Physicist and Coordinator for quantum sensing at CERN)
The curiosity drive of fundamental science and boundary conditions :
Dr. M. Doser said that fundamental science was a curiosity-driven exploration of the universe rather than an application- or goal-oriented exploration. The purpose of it was to obtain a better or new understanding of a particular topic. The process did not have a goal but was about the exploration itself. It was important to be open to serendipity and the role of random chance in the study of fundamental science. The hope was to uncover the unexpected.
Funding agencies tended to be reluctant to support fundamental science as it did not bring immediate societal benefits. There were, however, a great deal of long-term benefits that could be gained from fundamental science.
A number of “science ecosystem” boundary conditions must be met for fundamental science research to work. First, it was vital to accept that assumptions could be challenged and even overthrown. Second, multiple, redundant and different approaches might be needed which could cause wastage. Third, failure was an inevitable part of the process and the answers given might be incomplete, ambiguous and temporary. Fourth, severe criticism by other scientists was likely and even necessary to improve the work. Fifth, resource-limits meant that promising approaches might be dead-on-arrival while intellectual limits meant some answers might never be known. Consequently, the result of the research process was an imperfect, halting, iterative attempt at a better understanding. Funders needed to take the above conditions into account.
Science knew a great deal about the “Standard Model”, including quarks, protons, neutrons, nuclei, atoms, proteins, cells, planets, stars and galaxies. The knowledge was precise, tested and verified. However, very little was known about dark matter and dark energy, which represented 95% of the universe. The overall picture was therefore deeply incomplete. Major resources were needed to acquire more knowledge. The next steps went way beyond what any individual scientist, university or country could do, afford or justify. As such, it was necessary to take a global approach and move towards Big Science.
Big Science :
Big Science required major financial resources, major manpower, including expertise and motivation, as well as major energy resources. It also required long-term political, financial and moral support, commitment and stability over decades. In light of scarce resources, scientists would have to focus on a small number of questions. There would also be a need for multigenerational planning since the technologies took decades to develop. The idea would be for one generation to design the technology, the next to build it and the following to exploit it. Targeted streamlined engineering, rather than table-top-tinkering, was vital. Indeed, the engineering must be as cheap as possible, as reliable as possible, as redundant as possible and as fail-safe as possible. Equally, Big Science needed enhanced public and political dialogue, not the building of ivory towers, as well as an acceptance of a certain level of risk given that some experiments would inevitably fail. A decision must be made on how much risk was palatable. All of the above required democratic legitimacy within society, within national and global entities and within the scientific community itself.
Big Science meant focusing on a small number of big projects of the order of one billion euros or more. As such, some science targets would need to be dropped. To acquire democratic legitimacy, it was necessary to organize scientific priorities at the grassroots level. Every relevant stakeholder must be involved in the prioritization process so that all were on board with the final projects. Processes such as Snowmass and the European Strategy Group were already used to establish scientific priorities in the area of particle physics.
Given the vast amount of resources required, there was a need to build big communities around Big Science, which meant the projects would not be nimble. In other words, it would be difficult to change direction while keeping everybody on board. The lack of nimbleness posed an organizational and sociological challenge to collaborations.
Big Science was a rich country's pastime. For example, for every scientist engaged in particle physics, Austria paid about 100,000 euros per year for membership of CERN plus a further 100,000 euros per particle physicist per year to carry out experiments. It was an amount that was not accessible to every economy.
In addition, many projects, such as those that involved the construction of telescopes and accelerators, were likely to take decades. It could be that easier, simpler and quicker methods of doing the same thing were discovered along the way thus cutting down the timescale of the project, as had been the case with the Human Genome Project. Such discoveries were rare but could happen. It was therefore important to set aside money for new discoveries alongside the decadal projects.
The risks of Big Science :
There were a number of risks involved in Big Science. One risk was that some of the technology might become obsolete by the time a project was launched, as had been the case with space exploration. Rigidity was another big risk. It was also uncertain whether the interesting questions of today would be relevant tomorrow. Indeed, scientists were constantly discovering new fields and techniques which might overtake previous priorities. Nitrogen vacancy diamonds, for instance, was now a flourishing industrial field but had been completely unknown ten years previously. In addition, there were questions about how to keep scientists engaged in long-term projects given that much of the work was done by engineers. The risk of failure was also there as well as the need to focus on concrete, feasible questions rather than impractical ones. Lastly, there was a risk involved in terms of the compatibility of Big Science with the Sustainable Development Goals. The energy consumption at CERN had reached 1.4 TWh in 2022 but would go up to about 4 TWh if more colliders were put into operation. Four TWh was about one 150 thousandth of the energy used by all of humanity. It was important to ask whether such a high level of energy consumption was justified.
Small Science as an alternative :
The alternative to Big Science was Small Science, or fundamental science on a smaller scale. Small Science had great support due to its economic value. Many large countries and economic areas were investing Big Science budgets into numerous Small Science activities, particularly those linked to quantum technologies. The investments were of the order of approximately 10 to 100 billion euros over the next 5 to 10 years per country and aimed to inspire rapid societal impact and sociological change. Among the benefits of Small Science were its affordability and nimble nature as well as its ability to rapidly grow new technology within a few months or years. The risks, however, included duplication of efforts with many people working on the same areas, re-invention of the wheel and an inability to scale. It was also unclear whether it was possible to answer big questions, including those about dark matter, using small devices such as quantum sensors.
Six families of quantum sensors had been identified that were interesting to develop. The next step was to decide on where the technologies would be built. Activities were already going on in Europe, Asia and the United States of America. It would therefore be beneficial to create international collaborations. He warned against parochialism urging countries not to stay stuck in their own “playground”. There was also a need to take a dual approach, bridging fundamental research with applied science and creating devices that would be useful for both.
However, Small Science must also be made available to the rest of the world, including South America and Africa, which could still end up being expensive. There would be start-up and operation costs thus bringing the total amount up to about 100,000 euros per physicist. Disparities in educational backgrounds could also hamper efforts to expand in those regions. It was therefore important to improve the level of education across the globe.
Complementary approach between Big Science and Small Science :
He was in favour of a complementary approach between Big Science and Small Science which allowed for table-top fundamental physics alongside long-term Big Science projects. Global networks should be open from the get-go, allowing anybody to join and contribute with whatever resources they had. It would be an on-ramp for small institutions or economies, enabling them to participate without having to provide the hardware.
A complementary approach would require participants to balance priorities and split the funding. In his view, the optimal way to split the funding was to give 40% to Big Science, 40% to Small Science and 20% to blue-skies research. The split was in line with the concomitant failure rate that was acceptable for each area, namely 5% for Big Science, 30% for Small Science and 90% for blue skies research. It was necessary to play it safe when spending large amounts of money which meant that the failure rate of Big Science must be kept low. Blue skies research, however, must have a high in-built failure rate and funders must expect most of the money to be wasted.
Physicists must change their attitudes and come to see failure as good. It was through failure that solid ground could be built. There was also a need to diversify approaches as many would inevitably be unsuccessful. Diversity of communities was also important. By participating in research in concert with the global scientific community, countries would help educate their populations scientifically and technologically in a way that would have global impact but also bring national development.
In summary, fundamental science aimed to attract technical as well as curious individuals, foster creativity, and generally contribute to the welfare and cohesiveness of society. To do so, it must reflect societal changes, be open to and aware of the world in which it operated and be willing to build on scientists’ eagerness to interact. However, it also required an effort on the side of society to understand what science could offer, its limitations and needs. The same held true for global science, if anything more so, because collaborative and cultural aspects needed to be addressed flexibly. The latter point was simplified by the focus on fundamental rather than applied science.